Robotic Surgery Smart Materials Robotic Structures and Artificial Muscles 1st Edition by Mohsen Shahinpoor, Siavash Gheshmi – Ebook PDF Instant Download/Delivery: 9781498724531, 1498724531
Full dowload Robotic Surgery Smart Materials Robotic Structures and Artificial Muscles 1st Edition after payment
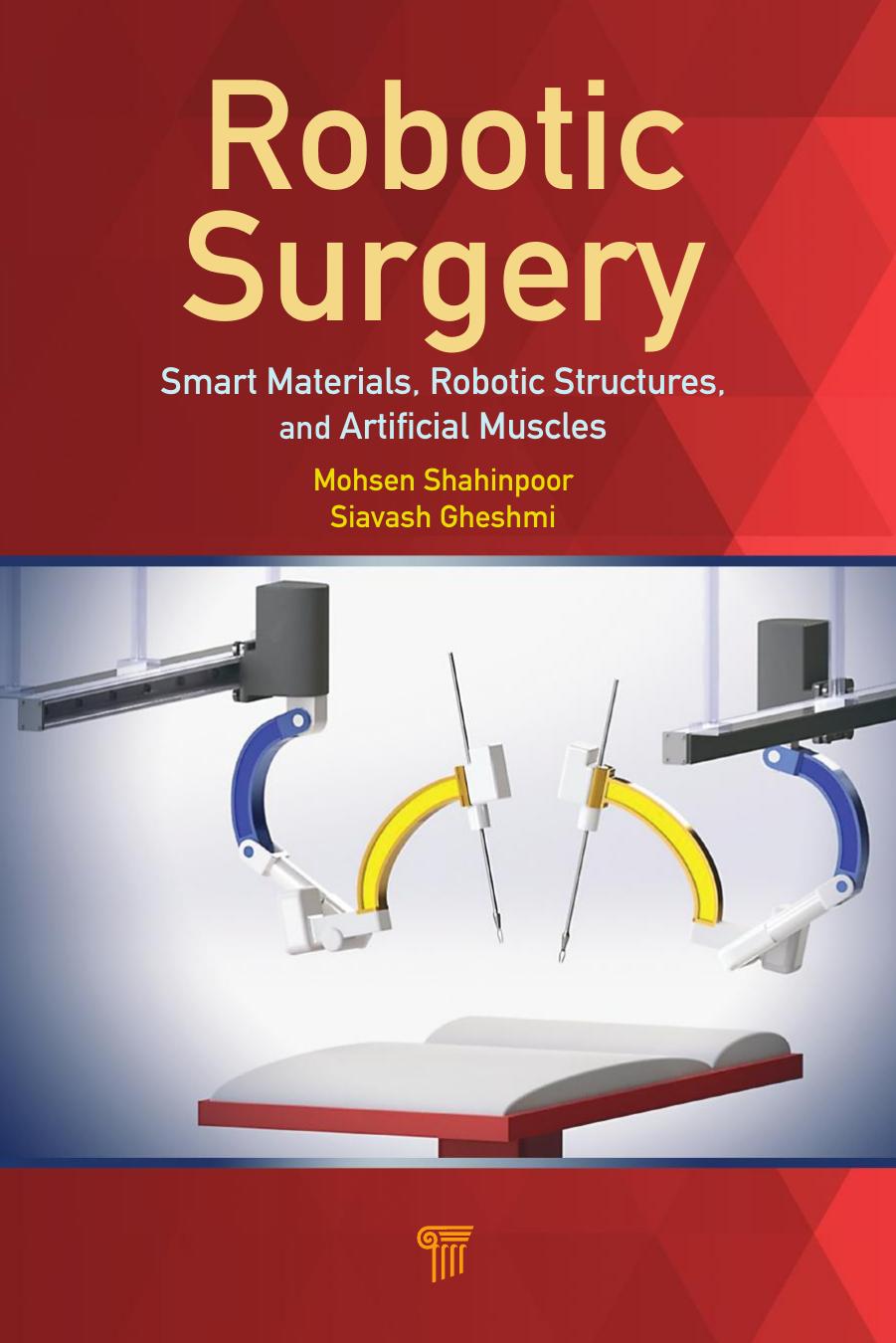
Product details:
• ISBN 10:1498724531
• ISBN 13:9781498724531
• Author:Mohsen Shahinpoor, Siavash Gheshmi
Robotic Surgery
Smart Materials, Robotic Structures, and Artificial Muscles
Robotic surgery has already created a paradigm shift in medical surgical procedures and will continue to expand to all surgical and microsurgical interventions. There is no doubt that in doing so robotic surgical systems, such as the da Vinci surgical system, will become smarter and more sophisticated with the integration, implementation, and syner
Robotic Surgery Smart Materials Robotic Structures and Artificial Muscles 1st Table of contents:
Chapter 1 Introduction to Surgical Robots’ General Configurations
1.1 History of Robotics
1.2 Introduction to Robotic Surgery
Figure 1.1 Compact laparoscopic surgical system by Ma and Berkelman (2007). (a) Arm components and (b) arms in practice.
Figure 1.2 The RAVEN surgical robot. (a) SolidWorks models and (b) arms in practice.
Figure 1.3 The robotic cameraman by Mirbagheri et al. (2011). (a) The robotic cameraman, (b) the cameraman in use, and (c) dexterity and workspace.
1.3 Robotic Systems in Human Surgery
1.3.1 Surgical Robotic Systems and Their Advantages
1.3.2 Applications of Robotics in Surgery
1.3.2.1 Neurosurgery
Figure 1.4 The PUMA 560 robot was first used in neurosurgery [Kwoh et al., 1988].
Figure 1.5 The SurgiScope robotic system. (a) SurgiScope set up in an operation room, (b) SurgiScope in use, and (c) a closer view of the system.
Figure 1.6 The NueroMate robotic surgical system. (a) A left-side view, (b) a right-side view, and (c) a closer view in operation mode.
1.3.2.2 Orthopedic applications
Figure 1.7 The ROBODOC surgical system.
Figure 1.8 The ACROBOT surgical system for knee replacement. (a) A side view of the system and (b) ACROBOT in use.
Figure 1.9 The CASPAR robotic surgical system.
1.3.2.3 Urology applications
Figure 1.10 The da Vinci® robotic surgical system.
Figure 1.11 The PAKY surgical robot.
Figure 1.12 The Probot surgical system. (a) A detailed view and (b) a general view.
1.3.2.4 Cardiovascular surgery applications
Figure 1.13 Robot-assisted microsurgery (RAMS).
Figure 1.14 Robotic mitral valve surgery via AESOP®7.
Figure 1.15 Proper patient position for robotic mitral valve surgery by Kypson, Nifong, and Chitwood (2003).
Figure 1.16 Cross section of the operative site in mitral valve surgery by Kypson, Nifong, and Chitwood (2003).
1.3.2.5 Gynecology applications
1.3.2.6 Ophthalmic surgery
Figure 1.17 The parallel robot for microsurgery by Dalvand and Shirinzadeh (2013).
1.3.2.7 Hair transplant
Figure 1.18 The ARTAS robotic surgical system. (a) A general view and (b) A RTAS® in use.
1.3.2.8 Other applications
Figure 1.19 Robotic neck surgery by Terris and Amin (2008).
Figure 1.20 Operative techniques in robotic-assisted laparascopic (a) cholecystectomy, (b) Nissen fundoplication, (c) gastric bypass, and (d) left hemicolectomy by Bochkarev, Ringley, and Oleynikov (2005).
Problem Set
Chapter 2 Surgical Robots’ Kinematics and Workspace
2.1 Kinematics of Robotic Manipulators
2.1.1 Introduction to Kinematics of Robotic Manipulators
2.1.2 General Description of Robotic Manipulators
Figure 2.1 Typical six-axis revolute robotic manipulator.
Figure 2.2 Relative configuration of the gripper and base coordinate frame in a robotic manipulator.
Figure 2.3 Definition of direct and inverse kinematics.
2.1.3 A Brief Description of Mathematical Notation
2.1.4 Mathematical Preliminaries on Vectors and Matrices
Figure 2.4 Description of the same point in two different coordinate frames.
2.1.5 Homogenous Representation of Points and Objects
Figure 2.5 Representation of homogenous vectors.
Figure 2.6 Object representation by means of homogenous transformation made up of the object’s homogenous characteristics.
2.1.6 Homogenous Transformations
Table 2.1 Common homogenous transformations
Figure 2.7 Object manipulation
2.1.7 Robotic Manipulator Joint Coordinate Systems
2.1.8 Denavit–Hartenberg Representations
Figure 2.8 General schematic description of robotic joints.
Figure 2.9 Generalized robotic link coordinate systems.
Figure 2.10 A cylindrical robotic manipulator.
2.1.9 Direct and Inverse Kinematics in Robotics
Figure 2.11 A two-link robotic manipulator.
Figure 2.12 A two-link robotic manipulator’s geometrical properties.
Figure 2.13 Two two-link robotic arms manipulating an object.
Figure 2.14 Geometrical properties of two two-link robotic arms.
2.2 Robotic Workspace
Figure 2.15 A 2D two-link robotic manipulator.
Figure 2.16 The two-link robotic manipulator workspace; case 1.
Figure 2.17 The two-link robotic manipulator workspace; case 2.
Figure 2.18 The three-link robotic manipulator workspace; case 1.
Figure 2.19 The three-link robotic manipulator workspace; case 2.
Figure 2.20 The four-link robotic manipulator workspace.
Figure 2.21 The 3D two-link robotic manipulator workspace.
2.3 Robotic Trajectory
Figure 2.22 Different tip trajectories of a three-link robotic arm under different joint parameter constraints.
Figure 2.23 Combined tip trajectories of the three-link robotic arm shown in Fig. 2.22, all illustrated in one picture for comparison.
Problem Set
Chapter 3 Intraocular Robotic Surgical System
3.1 Introduction to Ophthalmic Surgery
Figure 3.1 Right eye anatomy (viewed from above).1
Figure 3.2 Muscles of the left orbit.2
3.2 Robot-Assisted Intraocular Surgery
Figure 3.3 Robotic system developed by Meenink et al. (2010).
Figure 3.4 Robotic system developed by Sutherland et al. (2006). (a) A wireframe view and (b) the system in practice.
Figure 3.5 Robotic system developed by Ueta et al. (2009). (a) The system parameters and (b) the system in practice.
Figure 3.6 Steady hand manipulator developed by Mitchell et al. (2007); different representations.
Figure 3.7 Intraocular robotic surgery with the da Vinci® Surgical System.
3.3 Proposed Robotic Surgical System for Use in Ophthalmology
3.3.1 Introduction to Our System
Figure 3.8 The proposed robotic system for use in ophthalmic surgeries; different views.
Figure 3.9 The proposed robotic system for use in ophthalmic surgeries.
Figure 3.10 Degrees of freedom of the proposed surgical system.
Figure 3.11 The proposed robotic surgical system; a solid model.
Figure 3.12 The proposed robotic surgical system; a closer view of the robotic manipulators.
Figure 3.13 Typical surgical scenes employing the proposed robotic surgical system.
Figure 3.14 Typical surgical scenes employing the proposed robotic surgical system.
Figure 3.15 Typical surgical scenes employing the proposed robotic surgical system.
Figure 3.16 Typical surgical scenes employing the proposed robotic surgical system.
3.3.2 Forward and Inverse Kinematic Analysis
Table 3.1 D–H parameters specific to the proposed intraocular robotic surgical system
Figure 3.17 D–H coordinate systems on the proposed robotic surgical system.
Figure 3.18 A closer view of the D–H coordinate systems on the proposed system.
Figure 3.19 A closer view of the D–H coordinate systems on the proposed system.
3.3.3 Feasibility of Cataract Surgery with the Proposed System
3.3.3.1 Introduction to cataract surgery
Figure 3.20 Eye with (a) a clear lens and (b) a cataractous lens.3
3.3.3.2 Phacoemulsification with the proposed robotic system
Figure 3.21 Retrobulbar anesthesia.4
Figure 3.22 Dilated pupil.5
Figure 3.23 Anesthetic injection to different parts of the eye by the proposed robotic system.
Figure 3.24 Application of pupil-dilating eyedrops by the proposed robotic system.
Figure 3.25 Application of an eyelid speculum: (a) a lid speculum, (b) the lid speculum in use, (c) a lid speculum on our model
Figure 3.26 Paracentesis.
Figure 3.27 The proposed robotic system performing paracentesis.
Figure 3.28 The proposed robotic system injecting viscoelastic gel.
Figure 3.29 Capsulorhexis; different representations.
Figure 3.30 The proposed robotic system performing capsulorhexis.
Figure 3.31 Hydrodissection; different representations.
Figure 3.32 Hydrodelineation; different representations.
Figure 3.33 Hydrodissection and hydrodelineation.20
Figure 3.34 The proposed robotic system performing (a) hydrodissection and (b) hydrodelineation.
Figure 3.35 Phacoemulsification; different representations.
Figure 3.36 Phacoemulsification in practice.
Figure 3.37 The proposed robotic system performing phacoemulsification.
Figure 3.38 The structure of a common artificial IOL.26
Figure 3.39 Different commercial artificial IOLs.27
Figure 3.40 Implanting an artificial IOL.
Figure 3.41 An artificial IOL in place.30
Figure 3.42 The proposed robotic system implanting an artificial IOL.
Figure 3.43 The proposed robotic system removing viscoelastic gel.
Figure 3.44 Iridectomy with the proposed robotic system.
Problem Set
Chapter 4 Deployable Laparoscopic Robotic Surgical System
4.1 Introduction to Laparoscopic and Robotic Laparoscopic Surgery
Figure 4.1 Laparoscopic surgical procedure.
Figure 4.2 Laparoscopic and conventional cholecystectomy.2
4.2 AESOP®, ZEUS®, and da Vinci®: First Methods and Systems in Robotic Laparoscopy
4.2.1 AESOP®
Figure 4.3 AESOP® 3000 robotic surgical system; different views.
4.2.2 Zeus®
Figure 4.4 Zeus® robotic surgical system.
4.2.3 Da Vinci®
Figure 4.5 Da Vinci® robotic surgical system. (a) The whole system, (b) the surgical arm cart, and (c) the surgeon’s console.
Figure 4.6 The da Vinci® robotic surgical system in an OR.
Figure 4.7 The da Vinci® robotic surgical system main units. (1) A surgeon console, (2) image-processing equipment, (3) EndoWrist® instruments, (4) a surgical arm cart, and (5) a high-resolution 3D endoscope.
4.2.3.1 Surgeon console
Figure 4.8 The da Vinci® surgeon console; different views.
Figure 4.9 The da Vinci® surgeon console; different sections. (a) A surgeon touchpad, (b) a fingertip controller and scaling feature, and (c) foot pedals.
Figure 4.10 The da Vinci® surgeon dual console.
4.2.2.2 Image-processing equipment
Figure 4.11 The da Vinci® surgeon console vision system.
Figure 4.12 The da Vinci® image-processing equipment. (a) A wide-screen touch screen, (b) visual resolution (1080i HD), (c) a 3D HD camera head.
4.2.3.3 EndoWrist® instruments
Figure 4.13 Da Vinci® cannulas; different representations.
Figure 4.14 Da Vinci® EndoWrist® surgical instruments; different representations.
Figure 4.15 Da Vinci® EndoWrist® surgical instruments. (a) Degrees of freedom, (b) dexterity, and (c) instruments in use.
4.2.3.4 Surgical arm cart
Figure 4.16 The da Vinci® surgical arm cart.
4.2.3.5 High-resolution 3D endoscope
Figure 4.17 Da Vinci® high-resolution 3D endoscopes.
Figure 4.18 Difference between conventional surgical procedures and robot-assisted ones with the help of the da Vinci® robot.
Figure 4.19 Result of a robot-assisted surgery with the help of the da Vinci® robot.
4.3 Deployable Structures
4.3.1 Introduction to Deployable Structures
Figure 4.20 Linear deployment of the SLE mechanism.
Figure 4.21 The DNA structure one the left (schematically shown) will self-assemble into the structure visualized by atomic force microscopy on the right (Strong, 2004).
4.3.2 Geometrical Characteristics of Circular Deployable Structures
Figure 4.22 Circular deployable structure (a) in closed mode and (b) in deployed mode.
Figure 4.23 Dependency of the deployed radius on the offset and the amount of deployment.
Figure 4.24 Three-finger robotic grabber based on an SLE in deployed mode.
Figure 4.25 Four-finger robotic grabber based on an SLE in deployed mode.
Figure 4.26 An experimental model for a three-finger robotic grabber based on an SLE in deployed mode.
Figure 4.27 An experimental model for a four-finger robotic grabber based on an SLE in deployed mode.
4.3.3 Geometrical Characteristics of Curvilinear Deployable Structures
Figure 4.28 Initial curve, joint location, and path design.
Figure 4.29 Virtual modeling of the mechanism. (a) Deployed and (b) closed.
Figure 4.30 Trajectory of the end effectors vs. the actuation length.
Figure 4.31 Trajectory of the end effectors during deployment.
Figure 4.32 Horizontal displacement of the first three middle joints.
Figure 4.33 Vertical displacement of the last three middle joints.
4.3.4 Mathematical Model of Curvilinear Deployable Structures
Figure 4.34 Geometrical properties of a curvilinear deployable structure.
Figure 4.35 Change in the orientation of the last link vs. change in the displacement of the linear actuator.
Figure 4.36 Trajectory of the end joint for a 5.5 cm actuation.
4.3.5 Servo-Motorized Intermediate Pins: A Novel Approach
Figure 4.37 Novel deployable structure with motorized intermediate pins.
4.4 Laparoscopic Robotic System with Flexible Deployable Structures
4.4.1 Introduction to Our System
Figure 4.38 A typical linkage in an SLE structure with movable midspan pins.
Figure 4.39 The proposed space-efficient robotic surgical system; different views.
Figure 4.40 Different views from the surgical scene; flexible tubes installed around the deployable structure.
Figure 4.41 Application of covers on double-structure arms for enhanced safety.
4.4.2 Forward and Inverse Kinematic Analysis
Figure 4.42 Parameters of the proposed deployable robotic surgical arm.
Problem Set
Chapter 5 Applications of Smart Materials and Artificial Muscles in Robotic Surgery
5.1 Introduction to Smart Materials and Artificial Muscles
5.2 Applications of Ionic Polymer Metal Composites in Robotic Surgery
5.2.1 Brief Introduction to IPMCs as Multifunctional Materials
Figure 5.1 Typical deformation of strips (10 × 80 × 0.34 mm) of ionic polymers under a step voltage of 4 V.
Figure 5.2 Step response displacement characteristics of IPMNC samples (δ: arc length, Lo: effective beam length). Lo = 1.5 inches (bottom).
Figure 5.3 Displacement characteristics of an IPMC, ERI-S1 (δ: arc length, Lo: effective cantilever beam length). Lo = 1 inch (top, a) and 1.5 inches (bottom, b).
Figure 5.4 Variation of tip-blocking force and the associated deflection if allowed to move vs. the applied step voltage for a 1 cm × 5 cm × 0.3 mm IPMNC Pt-Pd sample in a cantilever configuration.
5.3 Feasibility of Providing Kinesthetic Force Feedback to Surgeons during Robotic Surgery by Electroactive Polymeric Sensors
Figure 5.5 A typical voltage response of an IPMC strip (1 cm × 4 cm × 0.2 mm) under oscillatory mechanical excitations. (a) Setup of cantilever configuration and (b) dynamic voltage output.
Figure 5.6 Cantilever and load cell configuration for measuring the tip-blocking force of IPMNC samples.
Figure 5.7 Typical sensing response of IPMC strips in bending. The sensing response is in millivolts, which can be correlated to the tip kinesthetic force
Figure 5.8 Experimental set-up for the measurement of bending force and curvature of IPMC cantilever strips using a high resolution load cell and impedance analyzer.
5.4 Integration of IPMCs with Robotic End Effectors for Kinesthetic Force Feedback to Surgeons during Robotic Surgery by Electroactive Polymeric Sensors
Figure 5.9 IPMC strips mounted on the face of grasping forceps (top and bottom) and wired in to the electronics.
Figure 5.10 Kinesthetic force feedback loop for haptic interaction with bodily organs.
Figure 5.11 Typical strong sensing signal in millivolts out of an IPMC strip in compression mode (direct compression of IPMCs with a normal load).
Figure 5.12 Typical force sensing signal in milli-Newton out of an IPMC strip (top: 10 × 30 × 0.2 mm, bottom: 10 × 30 × 0.4 mm) in bending/twisting mode (bending or twisting of IPMCs due to kinesthetic interaction).
Figure 5.13 Typical sensing signal in millivolts out of an IPMC in a loop configuration (loop deformation of IPMCs due to kinesthetic interaction of da Vinci® end effectors and plastic body organs). The voltage output can be correlated with kinesthetic normal force applied to the strip.
Figure 5.14 Some of typical da Vinci® surgical end effectors that can be integrated with various configuration of IPMCs for sensing the contact surfaces and kinesthetic forces experienced by surgeons during robotic surgery.
Figure 5.15 (a) Schematic of the experimental setup to measure the dynamic kinesthetic output voltage of IPMC strips in bending and electronically correlate it to the forces generated via torque measurements of the servo motor. (b) Ramp response of an IPMC sensor at four different curvature rates of 10 seconds, 20 seconds, 40 seconds, and 60 seconds. The IPMC strip was dynamically bent for an amount of 10 mm, which is equal to a 500 mm radius of curvature.
Problem Set
Chapter 6 Summary and Conclusions
Back Matter
Appendix A MATLAB Codes for the Generated Diagrams in Chapter 2
Fig. 2.16
Fig. 2.17
Fig. 2.18
Fig. 2.19
Fig. 2.20
Fig. 2.21
Fig. 2.22-a
Fig. 2.22-b
Fig. 2.22-c
Fig. 2.22-d
Fig. 2.22-e
Fig. 2.22-f
References
Author Index
Subject Index
People also search for Robotic Surgery Smart Materials Robotic Structures and Artificial Muscles 1st:
robotic surgery smart materials robotic
robotic surgery scholarly articles
robotic surgery software
robotic surgery studies
robotic surgery scar tissue
Reviews
There are no reviews yet.